VTU Module - 1 | Semiconductors
Module-1
- 4.9
-
2018 Scheme | ECE Department
- Created by VtuNotes.in
- 5 Modules
18EC33 | ELECTRONIC DEVICES | Module-1 VTU Notes
1. Bonding forces in solids:
Bonding forces in solids refer to the interactions that hold the constituent particles (atoms, ions, or molecules) together in a three-dimensional lattice structure, giving rise to the unique properties and behaviors of solid materials. Understanding these forces is fundamental in the field of materials science and plays a crucial role in various branches of chemistry, physics, and engineering.
There are primarily three types of bonding forces in solids:
- Ionic Bonding: In ionic solids, such as common table salt (sodium chloride), strong electrostatic forces attract positively charged cations to negatively charged anions, creating a stable crystal lattice. This type of bonding results in materials with high melting points, excellent electrical conductivity in molten or aqueous states, and brittle characteristics.
- Covalent Bonding: Covalent solids, like diamond and quartz, feature atoms that share electrons to form strong covalent bonds. These shared electrons are localized between adjacent atoms, forming a three-dimensional network. Covalent solids are known for their exceptional hardness, high melting points, and poor electrical conductivity.
- Metallic Bonding: In metallic solids, a sea of delocalized electrons flows freely among positively charged metal ions. This "electron sea" gives rise to metallic properties like malleability, ductility, electrical conductivity, and thermal conductivity. Metals also exhibit strong bonding forces, but the ability of electrons to move throughout the structure makes them unique.
The type and strength of bonding forces in a solid significantly impact its properties, including mechanical strength, electrical conductivity, thermal conductivity, and optical characteristics. By manipulating these forces, scientists and engineers can design materials with tailored properties for specific applications, ranging from semiconductors in electronics to superconductors in advanced technologies.
In summary, the study of bonding forces in solids is essential for unlocking the potential of materials, enabling innovation in various fields, and contributing to the development of new technologies that shape our modern world.
2. Energy bands:
Energy bands are a fundamental concept in the field of condensed matter physics and materials science, playing a crucial role in understanding the electronic properties of solids. Essentially, energy bands describe the distribution of energy levels that electrons in a solid material can occupy.
In a solid, such as a crystal lattice, the electrons are confined to specific energy states due to the interactions between atoms. Energy bands represent the quantized energy levels available to these electrons, with the lowest energy band, known as the valence band, containing electrons that are tightly bound to the atomic nuclei. Above it, there is an energy gap, called the band gap, which separates the valence band from the conduction band. In the conduction band, electrons have higher energy and are free to move throughout the material.
The behavior of electrons in these energy bands has profound implications for a material's electrical, thermal, and optical properties. Materials with a small or no band gap are typically good conductors of electricity, while those with a larger band gap are insulators. Semiconductors fall in between, with a moderate band gap that allows for controlled conductivity through the application of external factors like temperature or doping.
Understanding energy bands is vital for designing and engineering electronic devices, as it influences the flow of charge carriers, such as electrons and holes, which is essential for the operation of transistors, diodes, and other semiconductor devices. Overall, energy bands provide a foundational framework for exploring the electronic behavior and properties of materials in various scientific and technological applications.
3. Metals:
Metals are a diversified class of chemical elements with unique physical and chemical properties. They are important in research, engineering, and industry and make up a large part of the periodic chart.
Electrical circuits, power transmission lines, and heat exchangers require metals due to their high electrical and thermal conductivity. Their malleability and ductility allow them can be easily moulded into a variety of goods, from complex jewellery to building and bridge components.
Due to electron free mobility in their atomic structure, metals reflect light and have a metallic lustre. This particular electrical arrangement gives metals excellent density, durability, and toughness, making them essential in production and applications that require strength and resilience.
Ferrous and non-ferrous metals are usually classified. Iron and steel, which are iron-based, are utilised in construction, transportation, and manufacturing. However, non-ferrous metals like aluminium, copper, and gold are used in electronics, aerospace, and jewelry-making.
In addition to mechanical and electrical qualities, metals can undergo chemical processes like corrosion. Understanding and protecting these processes is crucial to the longevity and performance of metal-based products and structures.
Metals are essential to our civilisation, from our technologies to our infrastructure. Metallurgy, materials science, and chemistry help us comprehend metals and their many uses.
4. Semiconductors and Insulators:
Semiconductors and insulators are essential to modern technologies in materials science and electronics. These materials have electrical conductivity due to electron behaviour.
Semiconductors have qualities between conductors (metals) and insulators (rubber or glass). Semiconductors cannot conduct electricity, but they can under specific situations. This feature is used to make transistors, diodes, and integrated circuits, which power computers, smartphones, and solar panels. Silicon is the most extensively used semiconductor material.
In contrast, insulators aggressively resist electric current. A wide energy band gap means it takes a lot of energy to transport an electron from the valence band to the conduction band. Insulators include rubber, plastic, and ceramics. Insulating wires and cables, maintaining electrical safety, and preventing electrical interference require these materials.
Understanding semiconductors and insulators is essential for creating and engineering electronic devices and expanding telecommunications, renewable energy, and microelectronics technologies. The capacity to regulate and control these materials' electrical conductivity has changed modern life and communication.
5. Direct and Indirect semiconductors:
Modern electronics depend on semiconductors, which power microchips and solar cells. This category includes direct and indirect semiconductors. Electrons shift between energy bands in semiconductors, affecting their electrical and optical properties.
Direct Semiconductors:
In direct semiconductors, the bandgap between the valence band (where electrons are at lower energy levels) and the conduction band (where electrons may flow and conduct electricity) corresponds to photon energy. Electrons can easily transition from the valence band to the conduction band by absorbing or releasing photons with bandgap energy. Thus, direct semiconductors are useful for optoelectronic devices like LEDs and laser diodes due to their great optical absorption and emission.
Indirect Semiconductors:
Indirect semiconductors have a bandgap whose energy difference is not photon energy. Thus, electron transitions between the valence and conduction bands often entail phonon interactions. Electrons migrate less efficiently across energy bands through photon emission or absorption due to these interactions. Thus, indirect semiconductors have poorer optical characteristics than direct semiconductors.
Direct and indirect semiconductors must be distinguished in semiconductor device design and production. These features allow engineers and scientists to create optical and electrical components for transistors, photovoltaic cells, communication devices, and displays. This knowledge underpins semiconductor technological advances that form our networked world.
6. Electrons and Holes:
"Electrons and Holes" is essential to semiconductor physics and electronics. It refers to charge carriers in semiconductors, which are between conductors (metals) and insulators (rubber).
Electrons drive electrical conductivity in semiconductors like silicon. However, electron mobility in the crystal lattice structure creates "holes". When an electron moves between crystal lattice atoms, it leaves a "hole." This hole can act as a positively charged carrier without a particle. It describes the lack of an electron in a crystal lattice region.
Electrons and holes are essential to diodes, transistors, and integrated circuits. Designing and analysing electronic components requires understanding their behaviour. Electrons move in reaction to voltage or electric field, while holes move in the opposite way. Charge carrier movement is essential to electronic equipment and modern electronics technology.
In conclusion, "Electrons and Holes" is essential to understanding how charge carriers flow in semiconductor materials, enabling electronic devices and modern technology.
7. Intrinsic and Extrinsic materials:
Materials science divides substances into intrinsic and extrinsic types based on their properties and behaviour. These differences are essential for understanding and manipulating materials utilised in engineering, physics, chemistry, and electronics.
Intrinsic Materials: Pure materials, or intrinsic materials, are substances in their natural state. They have one type of atom or molecule, and their properties depend only on their elemental composition and crystal lattice configuration. Electrical, thermal, mechanical, and optical qualities are inherent to intrinsic materials. Copper, silicon, and diamond are inherent materials. Researchers research intrinsic materials to understand their properties and applications.
Extrinsic Materials: Intentionally modified or doped materials containing impurities to modify their properties. Adding foreign atoms or molecules to the material's crystal lattice structure can modify it. This lets engineers and scientists customise the material's properties for specific uses. Extrinsic materials include semiconductors like silicon doped with phosphorus or boron to improve electrical conductivity or ferromagnetic materials like iron with minor amounts of additional elements to optimise magnetic characteristics.
Understanding intrinsic and extrinsic materials is crucial in electronics, where semiconductors are crucial, and in material design for aircraft, automotive, and renewable energy. Researchers can create innovative technologies and products and learn more about the materials that influence our environment by manipulating these materials at the atomic and molecular level.
8. Conductivity and Mobility:
Conductivity and mobility are crucial to understanding materials' electrical behaviour in materials research and solid-state physics. Electronics, semiconductors, and energy storage devices require these ideas.
A material's conductivity is its electric current capacity. The presence and concentration of electrons and ions affect it. Materials with high conductivity allow electric charges to move smoothly, while those with poor conductivity hinder them. Conductivity is crucial to electrical component design and system performance.
Mobility measures how quickly charge carriers move in response to an electric field. It relies on carrier type (electrons or holes), temperature, and material impurities or flaws. Mobility is important for semiconductor device speed and efficiency. Higher mobility materials speed up electronic devices, improving integrated circuits and solar cells.
To develop new materials and technologies, researchers and engineers explore conductivity and mobility in academia and industry. Understanding these ideas allows the fabrication of sophisticated materials with specialised electrical properties, which is essential for meeting current technology's expectations. Conductivity and mobility are important topics for physics, materials science, and engineering students because they help develop sustainable energy solutions.
9. Drift and Resistance:
"Drift and Resistance" is a complex term with relevance across academic fields and society. It involves two opposing forces with different effects on change and stability.
Drift is the natural evolution of systems, ideas, and society in response to internal or external stimuli. It denotes a slow, sometimes unnoticeable change in status. Languages, ecosystems, and cultural norms drift.
Resistance is the opposing force that wants to keep things the same. This resistance might be caused by power structures, traditional values, or reluctance to adopt new ideas or practises. It counteracts drift to preserve systems and standards.
In academia, Drift and Resistance helps scholars analyse historical, sociological, political, and cultural issues. It helps us grasp change, continuity, and progress-enabling variables. Recognising drift and resistance helps explain complicated transformations in political ideology, technical advancements, and social movements.
Drift and Resistance can also be used to climate change, social justice, and technology. Universities and their students can better grasp our world's complex dynamics by researching how they interact, improving our understanding of the past, present, and future.
10. Effects of temperature and doping on mobility:
The "Effects of Temperature and Doping on Mobility" idea is essential to semiconductor physics and materials science, commonly studied at the university level. This topic examines how temperature and doping affect charge carrier mobility in semiconductor materials, such as electrons and holes.
1. Temperature: Charge carrier mobility in semiconductors depends on temperature. As temperature rises, crystal lattice vibrations (phonons) grow more energetic, dispersing charge carriers. Increased scattering reduces mobility, limiting the material's electricity conductivity. Understanding temperature-dependent mobility is crucial for building semiconductor devices that work across many temperatures.
2. Doping: To change a semiconductor's electrical characteristics, impurity atoms are purposely added. Doping can increase or decrease charge carrier mobility, depending on the dopant and concentration. P-type doping creates electron holes, while N-type doping adds electrons, increasing electron mobility. Dopant concentration also affects mobility, with higher doping levels causing dispersion and reduced mobility.
Optimising semiconductor devices like transistors, diodes, and solar cells requires studying how temperature and doping affect mobility. It lets engineers and researchers customise material electrical characteristics for energy-efficient devices or high-performance photovoltaic systems. This principle underpins sophisticated semiconductor physics and modern electronic technology.
11. Hall Effect:
In conductive materials, the Hall Effect occurs when an electric current travels through them in a magnetic field. The Hall Effect, discovered by American physicist Edwin Hall in 1879, is fundamental to electromagnetism and solid-state physics.
The Hall Effect causes a voltage difference (Hall voltage) between a conductor perpendicular to current flow and the magnetic field. Magnetic fields deflect charge carriers like electrons and holes, generating this voltage. As charge carriers experience a force perpendicular to their travel, they collect on one side of the conductor, causing a potential difference.
The Hall Effect is used to produce sensors for measuring magnetic fields, current, and semiconductor charge carriers. It also helps comprehend electron behaviour in conductive materials, advancing electronics, materials science, and semiconductor technologies.
Physicists and electrical engineers research the Hall Effect to understand how electric and magnetic fields interact in materials and systems. Its applications go beyond academia to automotive, aircraft, and electronics, where magnetic field and current measurements and control are crucial.
Course Faq
- Can we download the notes?
Yes, you can download the notes by going to the Module Topics and clicking on the View/Download Module Notes.
- How often notes are updated on AcquireHowTo?
We try our best to provide update notes to our users, so we keep updating them once a week.
- Do you provide only one specific university note?
No, Our team tries to work hard to provide notes from multiple universities like VTU, IP, DTU, Amity, etc, and from multiple courses like B.E, B.Tech, BBA, MBA, BCA, etc.
- Do the Notes you provide belongs to you?
No, the notes we provide belong to the only creator of that notes. May some note belongs to us but not all. AcquireHowTo is a notes providing platform that provide notes from different sources at one place to help the students.
Announcement
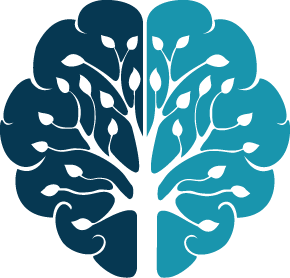
AcquireHowTo
Admin 1 year agoUpcomming Updates of the AcquireHowTo
- -- CGPA/SGPA Calculator with University Filter.
- -- Student Projects Guide and Download.
- -- Article Publishing platform for different categories.
- -- Courses for students on different topics.
- -- Student Dashboard for AcquireHowTo Products.
- -- Online Portal to buy Minor Projects and Major Projects.
- -- Last year Exams Question paper .
These all updates are comming soon on our portal. Once the updates roll out you will be notified.

COURSE INCLUDES

Maths Deptartment | 3rd Sem

CSE Deptartment | 3rd Sem

CSE Deptartment | 3rd Sem

CSE Deptartment | 3rd Sem

CSE Deptartment | 3rd Sem

CSE Deptartment | 3rd Sem

ECE Deptartment | 3rd Sem

ECE Deptartment | 3rd Sem

ECE Deptartment | 3rd Sem

ECE Deptartment | 3rd Sem

ECE Deptartment | 3rd Sem

ECE Deptartment | 3rd Sem

ECE Deptartment | 7th Sem

CSE Deptartment | 7th Sem

CSE Deptartment | 7th Sem

CSE Deptartment | 7th Sem

CSE Deptartment | 4th Sem

CSE Deptartment | 4th Sem

CSE Deptartment | 4th Sem

CSE Deptartment | 4th Sem
© copyright 2021 VtuNotes child of AcquireHowTo