VTU Module - 4 | Field Effect Transistors
Module-4
- 4.9
-
2018 Scheme | ECE Department
- Created by VtuNotes.in
- 5 Modules
18EC33 | ELECTRONIC DEVICES | Module-4 VTU Notes
1. Basic pn JFET Operation:
How a pn junction field-effect transistor (JFET) works is essential to understanding electronic devices and circuitry. In a JFET, 'p' symbolises the positive region (holes) and 'n' the negative region (electrons). This brief summary covers basic pn JFET operation:
- Gate-Source Voltage Control: The main idea of JFET operation is to control current flow between the source and drain terminals by altering the gate terminal voltage. Gate-source voltage (V_gs) controls channel conductivity.
- Depletion area: The channel's pn junction produces a depletion area when V_gs = 0. This depletion zone blocks electron and hole movement.
- JFET Modes: Enhancement and depletion modes operate. In enhancement mode, a negative gate-source voltage (V_gs < 0) increases the depletion zone, decreasing channel conductivity and drain current (I_d). Positive V_gs reduces the depletion zone, enabling greater current from source to drain.
- Pinch-off Voltage: The gate-source voltage at which the depletion area nearly closes and the drain current reaches its minimum is the pinch-off voltage (V_p). Beyond this voltage, the JFET is in the cutoff area, where I_d is almost nil.
- Transconductance (gm): JFETs can magnify modest gate-source voltage variations. It is calculated by dividing the change in drain current (ΔI_d) by the change in gate-source voltage (ΔV_gs). Transconductance is critical to JFET amplifier design.
- High Input Impedance: High input impedance is a major benefit of JFETs. For high-impedance input stages in electronic circuits, JFETs draw little input current due to the depletion region's insulation.
Basically, a pn JFET controls current flow between the source and drain terminals by altering the gate-source voltage. Designing electronic circuits that use JFETs for amplification and signal processing requires knowledge of JFET modes, pinch-off voltage, and transconductance.
2. Equivalent Circuit and Frequency Limitations:
Electrical engineering and electronics depend on "Equivalent Circuit and Frequency Limitations" to comprehend and create electronic systems. This notion states that complex electrical circuits using resistors, capacitors, and inductors can be simplified into an equivalent circuit that behaves the same but is simpler.
Engineering and research use equivalent circuits to analyse and analyse complicated systems and predict their behaviour under different conditions. Engineers can build and optimise circuits more effectively by reducing complex circuits to compute voltage, current, and power correlations.
Since electronic components have frequency-dependent behaviours, frequency restrictions are essential to this notion. Inductors and capacitors may self-resonate and have frequency-dependent impedance. These limits determine the frequency range where an equivalent circuit accurately replicates the original circuit.
Telecommunications, power electronics, and signal processing require comparable circuits and frequency limits. Engineers must examine these considerations when developing circuits for specific applications to ensure that the components and circuit topologies meet performance objectives across the frequency range.
Students learn to design and analyse comparable circuits, characterise frequency-dependent behaviour, and apply this knowledge to engineering challenges in university courses and research. Equivalent circuit analysis and frequency limits enable future engineers and researchers to create breakthrough electronic devices for today's technology-driven world.
3. MOSFET-Two terminal MOS structure- Energy band diagram:
The MOSFET is a basic electrical device used in integrated circuits and digital electronics. Energy band diagram analysis in a two-terminal MOS structure is essential to understanding its operation.
Two-terminal MOSFETs have a semiconductor substrate (typically silicon), an insulating SiO2 layer, and a metal gate electrode. The energy band diagram shows how charge carriers (electrons and holes) in certain materials affect device operation.
The energy band diagram shows electron energy levels in the semiconductor substrate, insulating layer, and metal gate electrode. The structure's cross-section displays these energy levels changing. A voltage applied to the gate electrode creates an electric field across the insulating layer, affecting semiconductor electron energy levels.
At the semiconductor-insulator interface, the "depletion region." forms an energy barrier or potential well. Controlling the gate voltage regulates the breadth and depth of this depletion region, which influences charge carrier flow between the source and drain terminals.
Designing and analysing MOSFETs in amplifiers, switches, and memory cells requires knowledge of the energy band diagram. It is essential to semiconductor device physics and integrated circuit design because engineers and researchers can optimise MOSFET performance.
4. Ideal Capacitance – Voltage Characteristics and Frequency Effects:
"Ideal Capacitance – Voltage Characteristics and Frequency Effects" is a key electrical engineering and electronics topic. Capacitors, passive electronic components that store electrical energy as an electric field between two conducting plates separated by a dielectric, behave this way. Designing and analysing electronic circuits requires knowledge of capacitor ideals and frequency behaviour.
The idealised behaviour of a capacitor is linear voltage across its terminals and charge storage. Thus, in ideal conditions, the voltage across a capacitor is directly proportional to its charge, as shown by the formula C = Q/V, where C is capacitance, Q is charge, and V is voltage.
Real capacitors may diverge from this ideal behaviour due to parasitic components and dielectric material defects. Deviations can cause frequency-dependent effects and non-linear capacitance-voltage characteristics.
As the applied voltage signal frequency changes, capacitors behave differently. Capacitors are ideal at low frequencies, but their impedance fluctuates with frequency. Frequency-dependent behaviour is measured by capacitive reactance (Xc), which is inversely proportional to frequency (Xc = 1 / (2πfC)), where f is frequency and C is capacitance.
Understanding capacitor frequency effects is crucial for electronic circuit filtering, signal coupling, and impedance matching. Engineers and researchers must consider these effects to build circuits that work well across frequencies.
In conclusion, "Ideal Capacitance – Voltage Characteristics and Frequency Effects" is crucial to electronic engineering. The optimal behaviour of capacitors and how their characteristics change with frequency form the basis for designing and analysing electrical circuits in many applications.
5. Basic MOSFET Operation- MOSFET structure:
Modern integrated circuits and electrical devices depend on the Metal-Oxide-Semiconductor Field-Effect Transistor, or MOSFET. Electrical and electronic engineering students must understand MOSFET operation and construction.
A MOSFET has a source, drain, and gate. Insulating layers divide these silicon substrate-based regions. The gate region is critical to source-to-drain current regulation. Voltage applied to the gate terminal creates an electric field in the semiconductor channel underneath. This field affects channel conductivity and current flow between source and drain terminals.
Two main types of MOSFETs are n-channel and p-channel. Electrons carry charge in n-channel MOSFETs and holes in p-channel ones. These devices operate and have various voltage polarities due to charge carrier differences.
Understanding Designing amplifiers, digital logic circuits, and power electronics need MOSFET operation. MOSFETs are essential in voltage amplification, switching, and energy-efficient electronic systems because engineers can accurately control current flow by varying the gate terminal voltage.
Modern electronics are based on MOSFET operation and construction. University students studying electrical and electronic engineering must understand these ideas to construct and analyse a variety of electronic systems and devices.
6. Current-Voltage Characteristics:
Current-Voltage I-V characteristics are essential to electrical engineering and physics. These properties explain the relationship between a device or component's electric current (I) and applied voltage (V). Analysing and developing electronic circuits, semiconductor devices, and electrical systems requires I-V knowledge.
The I-V curve of a device reveals its behaviour. It has unique patterns based on the component or material analysed. Consider these crucial points:
1. Ohm's Law: The most basic I-V characteristic is Ohm's Law, which asserts that a device's current is directly proportional to its voltage, with resistance as the proportionality constant. In this linear connection, resistance is constant across voltage.
2. Non-Ohmic Behaviour: Not all materials or components follow Ohm's Law. Many are non-Ohmic, featuring a nonlinear I-V curve that changes resistance with voltage or current. These include diodes, transistors, and semiconductors.
3. Diode Characteristics: Diodes have an I-V curve that enables current to flow in one direction (forward bias) but blocks it in the other. The curve shows the diode's threshold voltage and exponential current growth as voltage exceeds it.
4. Semiconductive Devices: The I-V characteristics of semiconductor devices like MOSFETs and BJTs are essential for developing integrated circuits and amplifiers. These components have complex, nonlinear curves that vary with operation.
5. Applications: I-V characteristics aid circuit analysis, troubleshooting, and design optimisation. Engineers utilise them to guarantee devices work safely and predict component behaviour in real-world applications.
6. Laboratory Measurements: University students studying electrical engineering or physics conduct experiments to detect and analyse I-V characteristics. These exercises reinforce theory and teach electrical skills.
Overall, Current-Voltage Characteristics explain how electrical components and materials react to voltage and current changes. This notion supports the design and operation of many electrical and electronic systems, therefore electrical engineers, electronics engineers, and associated professionals must master it.
Course Faq
- Can we download the notes?
Yes, you can download the notes by going to the Module Topics and clicking on the View/Download Module Notes.
- How often notes are updated on AcquireHowTo?
We try our best to provide update notes to our users, so we keep updating them once a week.
- Do you provide only one specific university note?
No, Our team tries to work hard to provide notes from multiple universities like VTU, IP, DTU, Amity, etc, and from multiple courses like B.E, B.Tech, BBA, MBA, BCA, etc.
- Do the Notes you provide belongs to you?
No, the notes we provide belong to the only creator of that notes. May some note belongs to us but not all. AcquireHowTo is a notes providing platform that provide notes from different sources at one place to help the students.
Announcement
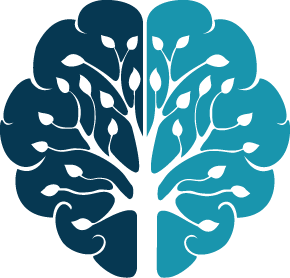
AcquireHowTo
Admin 1 year agoUpcomming Updates of the AcquireHowTo
- -- CGPA/SGPA Calculator with University Filter.
- -- Student Projects Guide and Download.
- -- Article Publishing platform for different categories.
- -- Courses for students on different topics.
- -- Student Dashboard for AcquireHowTo Products.
- -- Online Portal to buy Minor Projects and Major Projects.
- -- Last year Exams Question paper .
These all updates are comming soon on our portal. Once the updates roll out you will be notified.

COURSE INCLUDES

Maths Deptartment | 3rd Sem

CSE Deptartment | 3rd Sem

CSE Deptartment | 3rd Sem

CSE Deptartment | 3rd Sem

CSE Deptartment | 3rd Sem

CSE Deptartment | 3rd Sem

ECE Deptartment | 3rd Sem

ECE Deptartment | 3rd Sem

ECE Deptartment | 3rd Sem

ECE Deptartment | 3rd Sem

ECE Deptartment | 3rd Sem

ECE Deptartment | 3rd Sem

ECE Deptartment | 7th Sem

CSE Deptartment | 7th Sem

CSE Deptartment | 7th Sem

CSE Deptartment | 7th Sem

CSE Deptartment | 4th Sem

CSE Deptartment | 4th Sem

CSE Deptartment | 4th Sem

CSE Deptartment | 4th Sem
© copyright 2021 VtuNotes child of AcquireHowTo